Equipped with the latest, most accurate PID sensing technology from ION Science, the Ventis Pro5 from Industrial Scientific is now the most compact, versatile, and connected five-gas personal monitor available to reliably detect volatile organic compounds (VOCs). However, if you're considering adding the Ventis Pro5 with PID sensor to your fleet of gas monitoring equipment, there's a lot to consider about what's best for you and your facility—including why, how, what, where, and when to use this option for gas detection.
Join Ilaria Tramelli, business development director PID at Industrial Scientific, and Peter Morris, head of sensors at ION Science, as they discuss everything you need to know about PID technology.
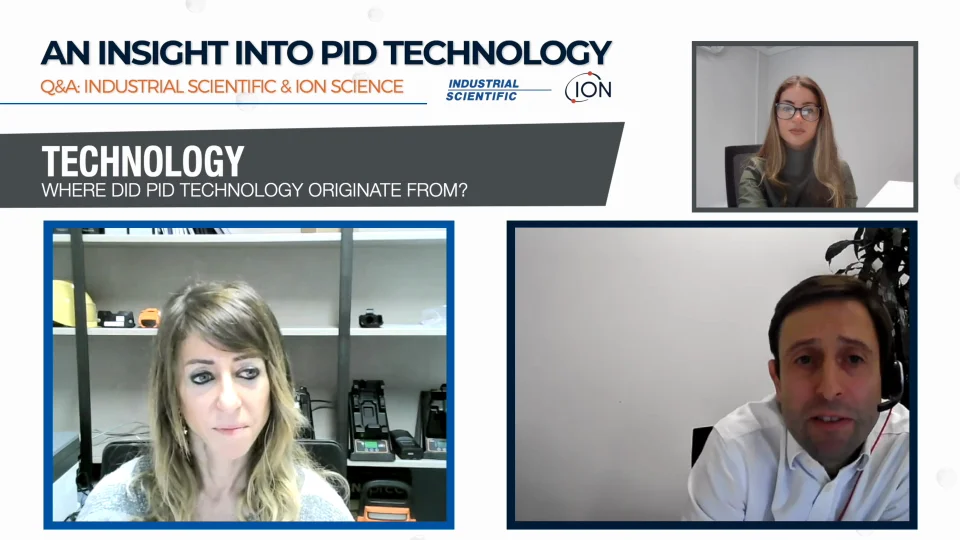
Interested in learning more about PID technology? Click here to watch the full conversation between Industrial Scientific and ION Science.
Video Transcript
Moderator: Can you advise on the technology available for monitoring VOCs?
Morris: There's many different techniques for measuring VOCs and they range in both costs and performance. You can buy a domestic VOC detector for a couple of hundred pounds or really high end, highly accurate laboratory equipment that can run into the hundreds or thousands.
The most common techniques for industrial health and safety and environmental monitoring are electrochemical, metal oxide, semiconductors, and PID. And they've all got their own advantages and disadvantages. Electrochemical sensors can measure VOCs down to ppm levels and the key advantages of electrochemical sensors are their low power consumption and relatively long lifetime. However, they do have disadvantages. They only respond to VOCs that are active, so it narrows the range of VOCs that it can monitor. They have a slow response time of approximately 90 seconds, so you can be alerted a little bit too late to a threat. And they're cross sensitive to other gases commonly found in industrial environments, including carbon monoxide, hydrogen sulfide and sulfur dioxide.
Metal oxide sensors can also measure VOCs down to ppm levels. And the key advantages of metal oxide sensors are they're low cost and they can have an extremely small form factor. The main disadvantages of them are their slow response time. Their response time varies depending on what VOC you're monitoring. The most rapid response time is about 60 seconds, but for bigger compounds it can be as low as 900 seconds. But the main disadvantage is that they're extremely sensitive to common gases, including NO2, NO, SO2, CO and CO2, and they're extremely sensitive to changes in humidity and suffer from long term drift.
PID is the most sensitive technique and can measure VOCs down to ppbs. So a few orders of magnitude more sensitive than the other techniques. Its main advantage is the response time. Its response time is a couple of seconds, and it's also the most selective technique to VOCs and also the most sensitive. And a real big advantage is that it's the most stable out of all the techniques and the environmental conditions that we see when we're monitoring industrial health and safety in our environmental environments.
One disadvantage is that it doesn't detect saturated hydrocarbons. The key sentencing attributes for detecting VOCs, for health and safety and environmental monitoring applications, are rapid response time, high sensitivity, and stability. And for these reasons, it's generally accepted PID is the best technique for measuring VOCs.
Moderator: Thank you, Pete. Please, could you go into a little bit more detail on how the PID works?
Morris: So it stands for photo ionization detection. That's quite a mouthful, so I'll try and break that down. Photo is Latin for light and ionization is the term used when a molecule gets broken down into positive and negative particles. So photo ionization is when light breaks down a molecule into a positively and negatively charged particle.
The main elements of a PID are a high energy light source, typically a low-pressure lamp containing krypton with a window that allows high energy light to pass through and an ionization chamber with electrodes to detect the charged particles. When VOCs enter the ionization chamber, light interacts with them and breaks them down into positively and negatively charged particles. And the negative particles are attracted to the positive electrode, and the positive ones are attracted to the negative. When they interact with the electrodes, it changes their current, and that change of current is proportional to the concentration of VOC present.
Emily: That's a great insight into the PID technology. Where did the technology first come from?
Morris: It was developed a while ago — initially in the 1960s by James Lovelock when he was working on electron capturing devices in Cambridge to measure CFC levels in the atmosphere. It was just one of the many brilliant things that he's done. Around this time we were starting to send people into space and thinking of exploring other planets and looking for life, and it was actually considered as a technique to be taken on the mission to Mars to detect signs of life.
The reason it was chosen is because it's relatively lightweight and low power, which were important considerations for space missions. The first development for a field PID occurred in the 1970s after Italian researchers reported vinyl chloride was a potential carcinogen. Soon after the Occupational Safety and Health Administration (OSHA) decreased the permissible exposure limits from 500 to 50 ppm. By 1974, the standard had decreased to 1 ppm and there were no portable analyzers available for measuring concentrations this low. A great person called Dr. Jack Driscoll founded a company called HNU to develop a PID to detect at these levels. And in the mid 1970s, the HNU 101 was released and it was quite a chunky device. If you think of a James Bond film back in the 60s and 70s, it's about the the size of a Geiger counter.
Towards the end of the 70s, the first mass field use of PID occurred. This was in the site investigation and cleanup of Love Canal. This was a residential development near Niagara Falls that had been built on an industrial landfill. It contained 63,000 tons of chemical processing waste. And after 10 years, residents were experiencing some serious health effects. The initial site investigation was done by taking soil samples and sending them to laboratories. However, there were so many samples being taken, there wasn't enough lab capacity to analyze them all and they needed a screening tool. So they're only sending samples that contain VOCs and the HNU 101 was employed to do this. This set PID as the standard for soil screening and created the first big market for PIDs and it's still the technique used for screening today. More recently, the technique has been miniaturized and power requirements reduced, and that has allowed wearable gas detectors to be developed.
Tramelli: And it's funny because I remember when I started my career 20 years ago, the HNU 101 was heavy, big, and it was really expensive at the time, and it was also very difficult to use. So you have to be trained to do that. But today, things are totally different. I mean, today a PID can be easily added to a standard personal gas monitor and that's the configuration. I would say that PID and LEL sensors are not two alternative technologies, but we should consider them as two complementary technologies. Our four standard gases plus PID should be a must have to protect workers from any kind of gas attack they face on the field in the short or long term.
Moderator: Historically there have been a few issues with using PID in certain environmental conditions. Can you explain what they are and how ION Science has overcome these issues?
Morris: One of the main issues with PID historically has been humidity and humidity affecting the reading, and it can cause two effects. It can cause readings to be attenuated, but it can also create false positives. So it's quite complicated pulling those two effects out, so I’ll try and go through that now.
When water vapor enters the ionization chamber, it will absorb UV light and reduce ionization efficiency. So there's lighter ionized VOCs. So what would happen if you were measuring 100 ppm of isoprenaline and you increased humidity? The response from your PID would drop from 100 to 60 ppm as humidity reached 99%. To overcome that, we've integrated a gas permeable, water impermeable membrane on the top of our sensor. This keeps most of the water out, but a little bit can pass through. So in addition to the filter, we've made our ionization chamber as thin as possible to minimize the amount of water vapor that can be present.
I'll switch to the second issue: false positives. False positives occur when fine grained contamination or photo ionization breakdown products deposit on the walls of the ionization chamber and electrodes. These fine particles are hydrophilic, and as humidity increases, they soak up water and can cause a short circuit. This can happen way below condensing humidity conditions. We've tackled this problem in two ways. Firstly, the filter keeps out fine particles, which limits contamination only to photo ionization breakdown products. Secondly, the membrane also retains ozone generated from the interaction of UV light with oxygen in the ionization chamber. That's a really efficient cleaning agent and that mops up any particles that sit down. So over time, and in extreme conditions, it is possible for contamination to build up and water can wet out on it. And to prevent this causing a short circuit, Ion Science has introduced a third electrode called the fence electrode and that mops up any breaker and keeps reading stable.
Moderator: Can temperature affect the performance of the MiniPID sensor?
Morris: Good question. Temperature affects the efficiency of electronics in general, and our sensor is no different. Typically, electronics run more efficiently when cold. So what we see in a standard PID, when it's cooler, the light would be brighter. When it heats up, the light would be dimmer and that would be apparent as a sensitivity drift as temperature changes. In many PID, we use an ASIC, an application specific integrated circuit, to monitor the sensor and manage it, and it gives really good temperature stability from -40C all the way up to 65C degrees. And by putting an asset in there, we managed to put the second benefit out. The asset monitors the status of the sensor and let the operator know if the lamp's not on or if the electricity and it helps provide failsafe assurance.
Moderator: Can any PID sensor detect a specific gas?
Tramelli: No, the sensor cannot detect a specific gas. The PID will respond to all the detectable volatiles present, and it's not possible to measure the concentration of each individual component. I would say that PID has to be considered as a screening tool that can immediately let you know if you are exposed to toxic levels of VOCs. If you wear a personal monitor with PID sensor close to your breathing zone, it will constantly check if toxic compounds are present in the air you are breathing, and if so, you should take some other actions such as to leave the area or to wear specific PPEs.
Interested in learning more about PID technology? Click here to watch the full conversation between Industrial Scientific and ION Science.